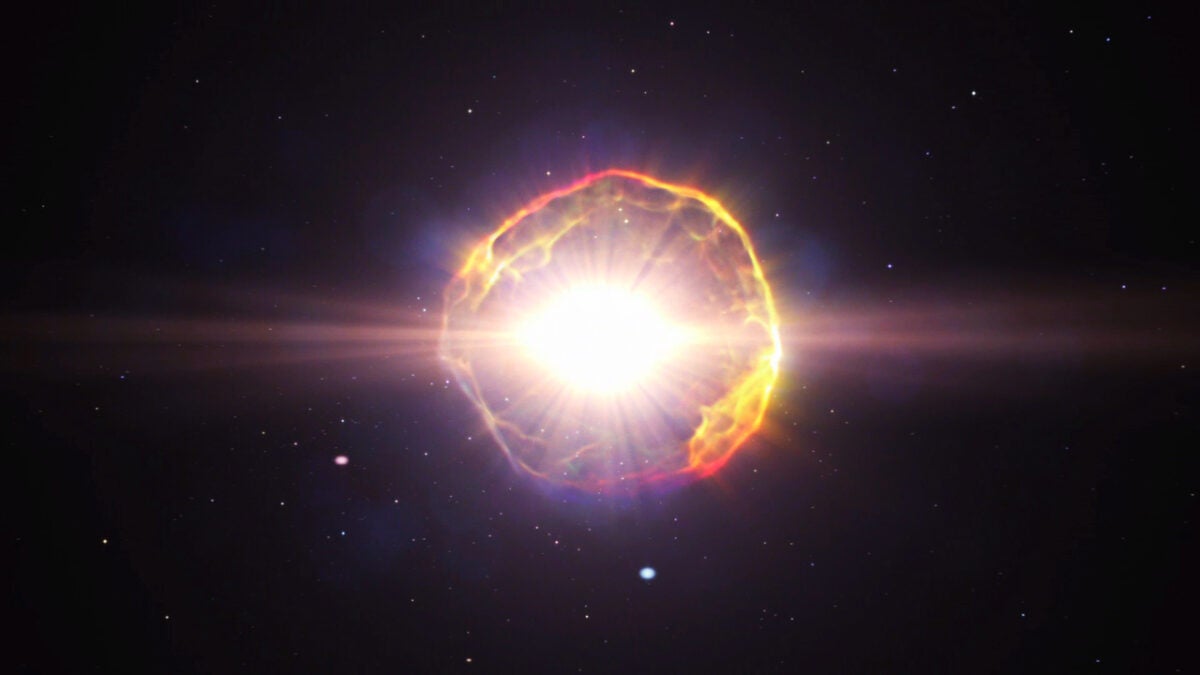
This still from an animation of NASA shows a star that goes in supernova. Credit: NASA’s Goddard Space Flight Center. Video courtesy of ESA/Hubble/L. Hearing
New research, published today in Astronomy of natureHe discovered that the water may have formed in the first 200 million years of the life of the universe. The vivifying molecule may have been created so quickly by the death of the first stars of the universe. The study also discovered that rocky planets could be built in the environment rich in water left behind, all even before the first galaxies existed.
The main investigator Daniel Whalen of Portsmouth University in the United Kingdom and his colleagues discovered that a rare type of supernova created only by the first stars formed enough water to soak the surrounding regions where the next generations of stars and their planets would be born.
The first stars
The very first stars were formed by hydrogen and Elio that filled the universe after the Big Bang. These were not in the galaxies – those did not yet exist – but instead to the crossings in which the filaments of Cobwilikes of the dark matter met, inserted between empty voids. Gravity attracted the gas on these crosses and when the density was quite high, the first stars were born.
These stars were huge, up to 300 times as much as our sun. Their temperatures were high and burned quickly through their fuel. And they died in supernovae who vomited new elements throughout the galaxy.
Whalen and his colleagues simulated two types of supernovae deemed prevalent between the first generation of stars following the life of the stars with 13 and 200 solar masses. “We looked at the primordial stars forming … and then they exploded,” says Whalen.
The first type of supernova is a supernova with the glue. These occur in stars at least eight to 10 times more enormous than the sun. With the age of the stars, they exhaust the hydrogen in their nucleus. Then they evolve through consecutive cycles of melting burning in a onion structure around the nucleus, merging increasingly heavy elements in new in a series of thin layers.
Iron is the last element created, because iron cannot be merged to create energy. So, in the current struggle between gravity and nuclear fusion (which creates photons that maintain the star), gravity finally wins and the core collapsed, creating a star of neutron, which cannot be further compressed. The rest of the star crashes; It affects the nucleus and rebounds, creating a shock wave. Inside the shock wave, it occurs even more fusion, this time creating heavier elements than iron.
At the same time, the intense pressures in the nucleus create neutrinos, which further energize the rebound material, eventually tearing the star. And all that material – including new formation metals (astronomers refer to any element different from hydrogen and helium as metals) – is launched outwards, leaving behind only the dense star nucleus.
Alternatively, the superneove of instability in couple occur only in stars well over 100 times the mass of the sun. The nuclei of these stars can reach the temperatures so hot that their photons turn into particles: pairs of electrons and positrons. The conversion of the energy on the matter abruptly reduces the pressure in the core so that suddenly it contracts. This releases enough energy to quickly kick more thermonuclear that translates into a shock wave that pieces the star to pieces.
The effect is like that of a huge bomb of hydrogen, says Whalen. The explosion is so powerful that the star does completely pieces, not leaving the nucleus back. Each trace of stellar material is launched outwards in space.
A supernova of instability in pairs can trigger up to 100 times the energy of an explosion of collapse of the core. “They were the first super powerful factories of heavy elements in the universe,” says Whalen.
After the explosion
What happened after the supernova surprised Whalen and his colleagues.
When the first stars exploded, they were surrounded by hydrogen gases remained by the star. Whalen’s simulations showed that the remaining material included small tufts linked together by gravity. While the hot ejecta of the supernova ran outwards, the metals inside – including oxygen – mixed with hydrogen, accelerating their gravitational collapse. The added metals also helped the tufts to cool, allowing oxygen to combine with hydrogen to form water.
However, things happened slightly differently in the two cases. While the material from a collective supernova of the core flowed relatively easily towards the outside, the material launched by a supernova of couples was chaotic, thanks to the most powerful explosion. The increase in turbulence in the second case has created more tufts and since the ejecta has not had to travel so far, it has also formulated significantly faster water. In addition, the highest pressure and temperatures of the supernova of instability in pairs have created material with multiple metals compared to the Corre-Collapse event, allowing the tufts to cool even faster.
The result is a significant temporal difference. While Core-Collapse supernovae form water within 30 million to 90 million years after their explosion, instability supernovas in couples can hydrate the surrounding environment in just 3 million years.
The amount of metals also made a huge difference. A Supernova Collapse Core creates only a few tenths of Solar Metal Massa, says Whalen. The superneove of instability per couple, on the other hand, approach 100 solar masses of metals, including 30-50 solar masses of oxygen. This can produce much more water.
The tufts around a collective supernova of the nucleus have become 10-30 times rich in water as clouds of gas spread in the Milky Way today. The superneove of instability per couple have also created richer groups, only a few times less rich in water than the sun system today.
Since they are more enormous, the stars that generate supernovae of couple instability have also had an advantage over their cousins. These are born, they burn and die in about 2.5 million years. The stars that produce supernovae Co-Collapse take more 12 million years to evolve. Therefore, the water was sown in the region around the most massive stars.
The first planets?
In both cases, the tufts left behind should form the next generation of stars. But what about the planets? There was a doubt that the planets could train from the debris created by the first stars. But some simulations suggest that these tufts could really be the site of the future formation of planets.
Guideo de Marchi, an astronomer at the European Space Research and Technology Center that was not involved in the new study, identified the protoplanetary records in environments similar to those of the early universe, in particular those with low metallicity (i.e. few heavy elements). In 2003, he says, Hubble found a huge planet in a globular cluster with very low metallicity.
The type of supernova could also affect the type of planets that are formed. The gas giants are mainly made up of hydrogen and helium in their external layers, while the rocky worlds need more silicate and other heavy elements to form. Since the material created by a supernova of instability in pairs has a higher metallicity (more complex elements) than that of a collective supernova of the core, the stars born from a central collective supernova have sufficient material to create planets similar to Jupiter, while the descendants of an explosion of instability per couple could be surrounded by rocky worlds.
And all this may have happened before the first galaxies are never formed.
Can we see it?
There is a warning: Whalen and his colleagues have explored only individual explosions. Most likely, several first generation stars would form together in which the cosmic filaments intersect. Each star would swim in the entire region in radiation, which has the potential to break the newly formed water molecules. At the same time, each region would also have dust, which would protect water from that radiation.
“It’s a delicate balance,” says De Marchi. But it remains optimistic. “The fact that we see the presence of water in the protoplanetary discs in low metallic environments [like] The small Magellanic cloud means that part of it can be preserved, “he says.
The hunt for the observable signs of the water in the initial universe remains a challenge. So also identify the first generation of planets around individual stars. Even the tufts of stars would shine too much to be identified with today’s tools. So Whalen and his colleagues are working to understand which type of report could produce those first stars and planets rich in water.
“An entire population [of water-rich stars and planets] In the first universe he could create this nebulous water … emission, “says Whalen. That issue could be potentially detected in the next decade by the great array of millimeters of Atacama in Chile or by the array of square kilometers under construction in Australia and South Africa.